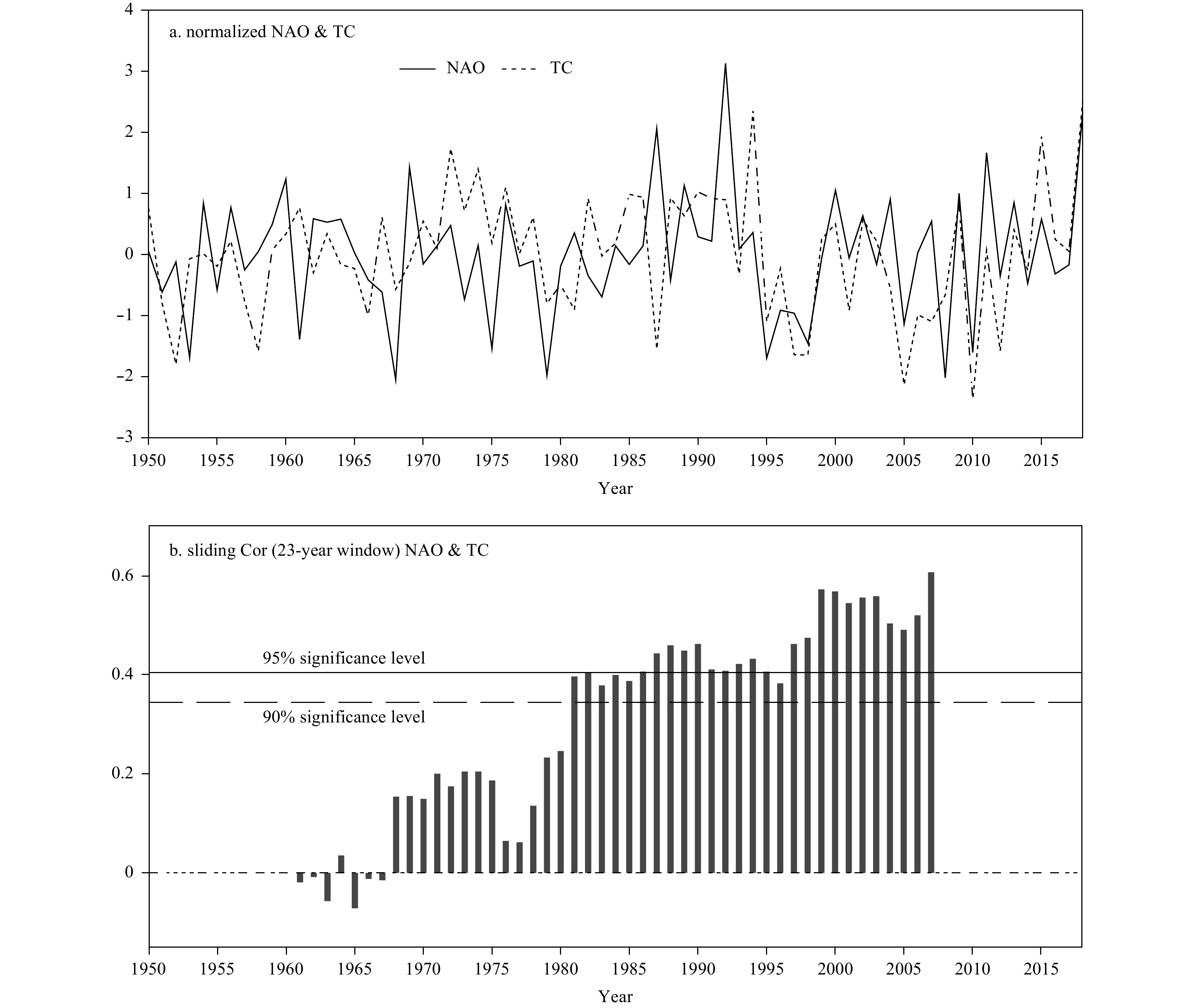
Citation: | Qun Zhou, Wen Chen. Unstable relationship between spring NAO and summer tropical cyclone genesis frequency over the western North Pacific[J]. Acta Oceanologica Sinica, 2020, 39(5): 65-76. doi: 10.1007/s13131-019-1509-0 |
The North Atlantic Oscillation (NAO) is the most dominant atmospheric mode in the North Atlantic region, and can be manifested by a sea level pressure (SLP) gradient between the Azores High and Iceland Low (Wallace, 2000). Many studies have documented the pronounced impacts of the NAO on the atmospheric circulation and climate over East Asia. The wintertime NAO exerts a significant influence on the East Asian winter monsoon (Wu and Huang, 1999), and may affect the precipitation of the following East Asian summer monsoon (EASM) (Sung et al., 2006) through the memory of the sea ice and sea surface temperature (SST) (Ogi et al., 2003). Several works have also reported the robust connection between the EASM and contemporaneous NAO (Sun et al., 2008; Sun and Wang, 2012). Recently, researchers have examined the time-lag relationship between the springtime NAO (especially that in late spring) and EASM (Gu et al., 2009a; Tian and Fan, 2012; Zuo et al., 2012), and have observed that the maintenance of the tri-pole SST anomalies in the North Atlantic associated with the spring NAO played a dominant role in this connection (Wu et al., 2009; Wu et al., 2011; Chen et al., 2019). Gu et al. (2009b) observed that the spring NAO-EASM relationship exhibited decadal variations which may be attributed to the change of anomalous SST pattern over the North Atlantic region. In addition, Zuo et al. (2012) further emphasized the effect of former winter NAO-SST anomalies tri-pole coupling mode on the North Atlantic tri-pole SST anomalies in the spring.
The western North Pacific (WNP) is the region with the most frequent tropical cyclone (TC) genesis in the entire world. Approximately one third of the total number of the world’s TCs occur in this basin (about 27 per year), which exerts disastrous impacts on the life and economy of people living in this region. A strong understanding of TC variability and its influencing factors may help to improve the seasonal prediction of TC activity, and thus is of great importance to society. Numerous works have analyzed the interannual variations of TC activity in the WNP region (Chen and Tan, 2010; Zhan et al., 2011). Previous studies have revealed that large-scale atmospheric circulation systems may be linked to the variations of the summertime WNP TC activity, such as the simultaneous Arctic Oscillation (AO) (Choi and Byun, 2010; Zhou and Zhang, 2018), Antarctic Oscillation (AAO) (Ho et al., 2005; Wang and Fan, 2007), Asian-Pacific Oscillation (APO) (Zhou et al., 2008), North Pacific Oscillation (NPO) (Wang et al., 2007), and NAO (Zhou and Cui, 2014). Evidence has shown that the preceding atmospheric teleconnection pattern may also serve as the predictor of TC activity over the WNP in the summer, such as the spring NPO (Chen et al., 2015), spring AO (Choi et al., 2012) and NAO in June (Choi and Cha, 2016). However, in view of the important role of the spring NAO in the East Asian summer climate, whether or not the spring NAO can influence the TC genesis frequency over the WNP in the following summer remains poorly identified. Moreover, the aforementioned relationships between the large-scale circulation patterns and WNP TC activity are unstable, and have undergone significant interdecadal variations in the past several decades (Zhou and Cui, 2014; Cao et al., 2015). This raises another interesting question: does an interdecadal change exist in the relation between the spring NAO and subsequent summer TC activity?
The main objectives of the present study are to address the above two issues, and to demonstrate the decadal variations in the spring NAO-summer TC relationship, so as to provide a better understanding of the changes in their interannual relationship may provide important information for the seasonal prediction of WNP TC activity. The remainder of this paper is organized as follows: The datasets and analysis methods adopted in this study are described in Section 2. Next, Section 3 presents the observational evidence for the interdecadal variations of the spring NAO-summer TC relationship. In Section 4, some possible reasons for the decadal changes are explored, based on the diagnosis analysis of the spring NAO-associated SST anomalies and the related physical processes. Finally, a summary of the main results of this study is given in Section 5.
In this study, the six-hourly TC best track dataset over the WNP region was obtained from the China Meteorology Administration (CMA), Tropical Cyclone Data Center. (
In this section, we provide evidence to demonstrate the fact that the impacts of spring NAO on TC genesis frequency in the subsequent summer over the WNP during the past several decades have not been stationary. Figure 1a shows the time series of normalized spring NAO index and following summer WNP TC genesis frequency index, both of which are characterized by significant interannual fluctuations. The correlation coefficient between these two indices for the entire period (1950–2018) is 0.29, which is statistically significant at the 95% confidence level based on the two-sided Student’s t-test. It is noteworthy that the relationship between these two indices appears to change during the study period with more opposite-sign values during the 1950s to late 1970s, yet with more same-sign values since the early 1980s to present. This suggests that the linkage between the spring NAO and summer TC genesis frequency is unsteady, and has exhibited interdecadal variations during the past several decades.
The interdecadal changes in this connection can be more clearly seen in the sliding correlations between the NAO index and TC genesis frequency index with a window of 23 years (23-year) (Fig. 1b). The years displayed in Fig. 1b are labeled according to the central year of the 23-year window. It is revealed that since 1981 (1997) the correlation coefficients are positive and significant above the 90% (95%) confidence level, based on the Student’s two-sided t-tests after calculating the effective degree of freedom, due to the reduced freedom degree (Cao et al., 2015). On the contrary, during the years before 1980 the positive correlations were much more greatly weakened, and before 1967 even negative correlations were observed. The results here confirm that the connection between the spring NAO and TC genesis frequency in the following summer over the WNP has exhibited an interdecadal shift around the early 1980s. The highest correlation coefficient appears in 2007 (i.e., 0.61), representing the central year of the 1996–2018 window. The lowest correlation coefficient occurs in 1965 (i.e., –0.07), corresponding to the window of 1954–1976 (Fig. 1b). As a result, we selected 1954–1976 and 1996–2018 as the two typical periods, with each period containing 23 years, and in the following sections of this paper we compare the related atmospheric circulations between the two epochs.
Earlier studies have shown that the TC genesis is controlled by the large-scale circulation and thermodynamical conditions (e.g.,Gray, 1968). Climatologically, the WNP monsoon trough extends along the southwestern periphery of the western Pacific subtropical high (WPSH), and thus the variations of the monsoon trough are closely related to the movement of the WPSH. Wu et al. (2012) pointed out that a strong WNP monsoon trough is accompanied by dynamic and thermodynamic factors that are favorable for the occurence and development of TCs, while a weak monsoon is not. Therefore, we first compare the NAO-related summertime horizontal winds at 850 hPa and geopentential height at 500 hPa in both the former and latter epochs in Fig. 2. We observed notable differences in both the lower and middle troposphere between the two periods. During the period of 1996–2018, a low-level cyclonic circulation anomaly was located in the tropical WNP region, whereas an anticyclonic circulation anomaly occupied the region east of Japan (Fig. 2b). This signifies that when the spring NAO is positive, there is an anomalous cyclone covering the tropics in the following summer, thus leading to an intensified WNP monsoon trough that is advantageous for TC genesis (Wu et al., 2012). At 500 hPa, a similar pattern is found with significant negative values appearing in the South China Sea and east of the Philippines, while relatively weaker but positive anomalies are observed to the northeast. This indicates that the summertime WPSH tends to be much weaker and retreats northeastward, corresponding to a positive spring NAO (Fig. 2d). Therefore, the enhanced WNP monsoon trough combined with the weakened and northeastward shifting WPSH during the positve NAO phase is in strong agreement with the increasing total TC genesis number in the subsequent summer during the period of 1996–2018. In contrast, when the spring NAO is negative, the WNP monsoon trough becomes weaker than normal and the WPSH is stronger and extends more southwestward, both of which are disadvantageous for the summer TCs formation during the period of 1996–2018. However, during 1954–1976, in comparison, the spring NAO-associated atmospheric circulation anomalies at the lower-level and mid-level atmosphere in the following summer are fairly weak and insignificant, thus suggesting that the spring NAO does not have a substantial influence on the WNP monsoon trough and WPSH in the following summer (Figs 2a and c). Consequently, the NAO-WNP TC connection is remarkably weakened in the period of 1954–1976 compared to 1996–2018.
Many studies have documented that environmental factors, such as low-level vorticity, middle-level relative humiduty, vertical wind shear and so forth, exert significant impacts on the formation of the TCs over the WNP region (Cao et al., 2015; Zhang et al., 2018; Zhou et al., 2019). Therefore, we further compare the differences of the dynamic and thermodynamic conditions closely associated with TC genesis between the two subperiods. Figure 3 shows the regressed distributions of the SLP, vertical velocity at 500 hPa, vertical zonal wind shear (between 200 and 850 hPa) and relative humidity (700–500 hPa averaged) anomalies on the spring NAO index in the two periods. Noticeable differences can be observed between the two epochs. During the period of 1996–2018, nearly the entire WNP region was dominated by significantly below-normal SLP and 500-hPa vertical velocity (Figs 3b and d). Moreover, the vertical zonal wind shear between 200 and 850 hPa are evidently reduced south of 15ºN, whereas the middle troposphere relative humidity was greatly enhanced during the active TC seasons over the tropical WNP (Figs 3f and h). These results imply that strengthened lower-level cyclonic vortivity, intensified vertical motion, decreased vertical zonal wind shear and increased relative humidity are present in a positive spring NAO year, all of which correspond well with the growing number of summer TC genesis over the WNP during the period of 1996–2018. Nevertheless, during 1954–1976, the anomalous SLP, vertical motion, vertical wind shear and relative humidity over the WNP are insignificant (Figs 3a, c, e and g), which is in accordance with the poor linkage between the spring NAO and summer TCs over the WNP region. As a result, the diagnosis of the large-scale dynamic and thermodynamic factors over the WNP here seems to support the fact that the springtime NAO may impose a much stronger influence on WNP TC genesis frequency in the following summer during 1996–2018 than 1954–1976.
In this section, we answer the question of why the spring NAO-related large-scale atmospheric circulation anomalies over the WNP in the following summer are different between the two subperiods. In addition, we investigate the possible mechanism responsible for the interdecadal change of the spring NAO-summer TC genesis relationship. Previous studies have shown that the NAO-induced tri-pole SST anomalies over the North Atlantic play a crucial role in the time-lag relationship between the springtime NAO and following EASM (Wu et al., 2011; Zuo et al., 2012). Accordingly, we plot the correlation patterns between the spring NAO index and the contemporaneous ones, as well as subsequent summer SST anomalies for the two epochs (Fig. 4). During the period of 1996–2018, the spring NAO index is highly correlated with the spring SST anomalies over most parts of the North Atlantic Ocean with the apparently warming SST located in the middle latitudes and cooling SST in the tropics and high latitudes, in association with a strong NAO event (Fig. 4b). Additionally, the similar anomalous SST tri-pole pattern could be clearly seen in the following summer with the warming areas slightly smaller in size and the cooling areas significantly enlarged (Fig. 4d). In contrast, during 1954–1976, the spring and summer SST anomalies related to the spring NAO were almost insignificant throughout the entire North Atlantic region (Figs 4a and c). The analysis results suggest that there is no robust relationship between the NAO and North Atlantic SST anomalies in the former epoch, while the tri-pole SST anomalies pattern is firmly established in the spring and may persist into the subsequent summer in the latter epoch.
A previous study by Wu et al. (2011) revealed that the establishment and development of this tri-pole SST anomaly pattern may be partially attributed to the variations of the atmospheric circulation and latent heat flux over the North Atlantic. Therefore, we examined the climatological circulation field and circulation anomalies from the spring to summer under the impacts of the spring NAO (Figs 5 and 6). During 1996–2018, the spring NAO exerted a great impact on the simultaneous 850 hPa wind field. Above-normal latent heat flux (LHF) anomalies cover the subtropics and the region north of 40°N over the North Atlantic, where anomalous winds are in the same direction as multi-year mean winds in the lower troposphere. The below-normal LHF anomalies are observed along the coast of North America in the subtropics, which could be attributed to the advection of warm air from the south (Figs 5b, d and f). This indicates that, due to wind-evaporation effects, the western subtropical North Atlantic absorbs heat from the atmosphere while the ocean releases the heat into the atmosphere on its poleward and equatorward side, thereby resulting in the formation of a prominent tri-pole SST anomaly pattern during 1996–2018 (Fig. 4b), which closely corresponds with the results of the previous study by Wu et al. (2011). In the subsequent summer, the NAO-related 850-hPa wind anomalies tend to be greatly reduced. The resulted LHF signals are remarkably weakened with the positive (negative) LHF anomalies appearing in the warmer (cooler) western subtropics (tropics and higher latitudes) over the North Atlantic (Figs 6b, d and f). This LHF anomaly pattern suggests the forcing of the SST on the atmosphere in the summer, and also favors the persistence of tri-pole SST anomalies, since the anomaly values are weaker than those in the spring.
However, compared with those in 1996–2018, both the springtime and summertime atmospheric circulation and latent heat flux anomalies become much weaker in the former period of 1954–1976 (Figs 5a, c, e; Figs 6a, c and e), which is in agreement with the hardly significant SST patterns in Figs 4a and c. Therefore, the diagnosis results of this study support the fact that the formation and sustenance of the NAO-related tri-pole SST anomaly may be attributed to the change of the LHF over the North Atlantic, thereby leading to a poor (robust) NAO-SST relationship during 1954–1976 (1996–2018).
It is noteworthy that the climatological summertime lower tropospheric wind field is characterized by an anticyclone in the mid-latitudes over the North Atlantic and a cyclone in the subtropical plateau area over Eurasian continent during both the former and latter epochs (Figs 6a and b). In fact, in order to facilitate the further analysis of the atmosphere circulation influenced by the ocean, we calculate the composite differences of the summertime circulation throughout the troposphere between the two subperiods. The results show that there are no evident teleconnection patterns across the North Atlantic-Eurasian continent (figures not shown). Therefore, the different responses of the circulation to the North Atlantic SST anomalies (Figs 7 and 8) in the summer between the periods of 1954–1976 and 1996–2018 may not result from the change in the mean circulation. In order to better understand large-scale circulation features coupled with the North Atlantic SST anomalies, further examinations are performed by calculating the summer 200 hPa westerly jet and geopotential height anomalies regressed on the summer tri-pole SST index (Fig. 7). The summertime North Atlantic tri-pole SST index is defined as the normalized difference of the averaged SST in the above-normal correlation box, minus the sum of the averaged SST in the two below-normal correlation boxes on each side in Fig. 4d. Therefore, Fig. 4d illustrates the positive phase of the tri-pole SST index with warmer SST anomalies in the middle latitudes and cooler SST anomalies in both the lower and higher latitudes in the North Atlantic Ocean. In Fig. 7b, a remarkable zonal propagating wave-train can be observed during 1996–2018, which originates from the North Atlantic toward the North Pacific along the northward flank of the westerly jet. The negative anomalies cover the North Atlantic, Ural Mountain and Sea of Japan, whereas the positive anomalies are found in the Western Europe and Lake Baikal regions. Moreover, the East Asian jet stream seems to be strengthened above the region east of Japan, which is a result of the anomalous westerlies along the south of the cyclone centered in the Sea of Japan. During the period of 1954–1976, the above subpolar eastward-propagating teleconnection pattern over the northern Eurasian continent becomes obscure, while the East Asian jet flow tends to be weakened compared with that during 1996–2018 (Fig. 7a).
Next, we further investigate how the anomalous atmospheric circulation over the extra-tropics in the WNP modulates the TC activities, especially in terms of the anomalous convection in the tropical region. Figure 8 shows the regression patterns of the vertical motion anomalies over the WNP (120°E–180°) on the summer North Atlantic tri-pole SST index for the two subperiods. During 1996–2018, the local Hadley circulation is characterized by a distinct descending motion in the subtropics, while significant ascending motions are observed on its north and south sides, with the most striking rising branch in the tropics south of 20°N (Fig. 8b). These observations imply that the deep convection over the WNP tropical region may be enhanced during the positive phase of the tri-pole SST anomalies, which is conducive to the genesis of TCs over the WNP. Conversely, during the period of 1954–1976, the summertime anomalous meridional circulation over the WNP in relation to the tri-pole SST index becomes evidently reduced. The descending branch in the extra-tropics in the Northern Hemisphere is extremely weak, and hardly significant. The ascending branch in the tropics is much weaker and confined below 300 hPa, thereby breaking the linkage of the deep convection over the WNP and the North Atlantic tri-pole SST pattern (Fig. 8a).
The above analysis suggests that the spring NAO-induced tri-pole SST anomalies pattern over the North Atlantic, persisting from spring to the subsequent summer, may have a fundamental effect in the connection between the spring NAO and summer upper troposphere jet anomalies in the downstream over the East Asian region. Previous studies have shown that the East Asian westerly jet plays an important role as a link between the EASM and the NAO (Tian and Fan, 2012; Zuo et al., 2012). The results of the present study further emphasize the fact that the changes in the local meridional circulation over the WNP may serve as a bridge in linking the extra-tropical atmospheric signals to those over the tropical areas. The East Asian jet flow is intensified (weakened) resulting from an eastward-propagating wave-train across the northern Eurasian continent forced by the North Atlantic anomalous SST tri-pole when the NAO is positive (negative) during the latter epoch. In addition, the jet stream variations further provide favorable (unfavorable) environmental factors for TC genesis over the WNP, via the modulations of the local meridional circulation. However, during the former epoch, the spring NAO-summer TC relationship becomes insignificant, due to the weak connection between the NAO and the SST anomalies over the North Atlantic region (Fig. 9).
Previous studies have indicated that the summer/June NAO had a significant influence on the simultaneous/July-August TC activities over the WNP region (Zhou and Cui, 2014; Choi and Cha, 2016). Regarding the change of the relationship between EASM and the preceding spring NAO (Wu et al., 2011; Zuo et al., 2012), this study presents evidence for an interdecadal change in the connection between the spring (April–May mean) NAO and the following summer (June–September) TC genesis frequency over the WNP, based on the NCEP-NCAR reanalysis data. Due to the fact that the NCEP-NCAR reanalysis may include some biases due to the changes in the amount of observational data assimilated into the reanalysis system (Yang et al., 2002; Wu et al., 2005), the ERA40 reanalysis is also used to re-examine the NAO-TC relationship and draw a similar conclusion (Figures not shown). The results included here demonstrate that the NAO-TC relationship is weak and insignificant before the early 1980s, yet strong and significant after the early 1980s.
Next, to explore this interdecadal variation of the spring NAO-summer TC relationship, we compared the spring NAO-associated dynamic and thermodynamic conditions, SST anomalies and physical processes between the two subperiods of 1954–1976 and 1996–2018. During the latter epoch, a pronounced cyclonic anomaly appeared in the tropical WNP region, and the WPSH tended to be much weaker in the summer when the spring NAO was positive. The strengthened monsoon trough resulted in an intensified low-level vorticity, decreased vertical wind shear, increased vertical velocity, and enhanced mid-level relative humidity, all of which were advantageous for TC formation and development over the WNP region. On the contrary, when the spring NAO was negative, the monsoon trough was weakened with the intensified WPSH extending southwestward, thereby leading to a higher SLP, stronger vertical wind shear, weaker vertical motion, and reduced relative humidity, which suppressed the TC genesis frequency during the summer. During the former epoch, the spring NAO-related large-scale environmental factors anomalies were hardly significant in the summer over the WNP region, thereby confirming the weak phase of the spring NAO-summer TC relationship.
The atmospheric circulation responses in the WNP in the following summer can be attributed to the maintenance of the North Atlantic tri-pole SST anomalies pattern induced by the spring NAO. During the period of 1996–2018, the anomalous tri-pole SST pattern was highly established, and may have persisted into the following summer due to wind-evaporation effects. The East Asian westerly jet at the upper troposphere was enhanced, corresponding to a positive spring NAO via a tri-pole SST-associated teleconnection wave-train over the subpolar region from the North Atlantic to the North Pacific. Through the variations of the local Hadley circulation, the deep convection in the tropical WNP was further strengthened, resulting in an increasing total TC genesis number over the WNP. This can effectively explain the in-phase linkage between the spring NAO and TC genesis frequency over the WNP in the following summer during 1996–2018. In comparison, during 1954–1976, the NAO-induced North Atlantic SST anomalies tri-pole was inconspicuous, thereby resulting in an insignificant influence of the spring NAO on the following summer circulation anomalies and TC genesis frequency over the WNP basin.
[1] |
Cao Xi, Chen Shangfeng, Chen Guanghua, et al. 2015. On the weakened relationship between spring Arctic Oscillation and following summer tropical cyclone frequency over the western North Pacific: A comparison between 1968–1986 and 1989–2007. Advances in Atmospheric Sciences, 32(10): 1319–1328. doi: 10.1007/s00376-015-4256-y
|
[2] |
Chen Guanghua, Tam C Y. 2010. Different impacts of two kinds of Pacific Ocean warming on tropical cyclone frequency over the western North Pacific. Geophysical Research Letters, 37(1): L01803. doi: 10.1029/2009GL041708
|
[3] |
Chen Dong, Wang Huijun, Liu Jiping, et al. 2015. Why the spring North Pacific Oscillation is a predictor of typhoon activity over the Western North Pacific. International Journal of Climatology, 35(11): 3353–3361. doi: 10.1002/joc.4213
|
[4] |
Chen Wen, Zhou Qun, Xue Xu. 2019. Solar cycle modulation of the relationship between the boreal spring Northern Atlantic Oscillation and the East and Southeast Asian summer climate. Meteorology and Atmospheric Physics, 1: L01803–9. doi: 10.1007/s00703-019-00687-4
|
[5] |
Choi K S, Byun H R. 2010. Possible relationship between western North Pacific tropical cyclone activity and Arctic Oscillation. Theoretical and Applied Climatology, 100(3–4): 261–274
|
[6] |
Choi J W, Cha Y. 2016. Possible relationship between NAO and tropical cyclone genesis frequency in the western North Pacific. Dynamics of Atmospheres and Oceans, 77: 64–73. doi: 10.1016/j.dynatmoce.2016.08.006
|
[7] |
Choi K S, Wu C C, Byun H R. 2012. Possible connection between summer tropical cyclone frequency and spring Arctic Oscillation over East Asia. Climate Dynamics, 38(11–12): 2613–2629
|
[8] |
Gray W M. 1968. Global view of the origin of tropical disturbances and storms. Monthly Weather Review, 96(10): 669–700. doi: 10.1175/1520-0493(1968)096<0669:GVOTOO>2.0.CO;2
|
[9] |
Gu Wei, Li Chongyin, Li Weijing, et al. 2009a. Interdecadal unstationary relationship between NAO and East China’s summer precipitation patterns. Geophysical Research Letters, 36(13): L13702. doi: 10.1029/2009GL038843
|
[10] |
Gu Wei, Li Chongyin, Wang Xin, et al. 2009b. Linkage between Mei-yu precipitation and North Atlantic SST on the decadal timescale. Advances in Atmospheric Sciences, 26(1): 101–108. doi: 10.1007/s00376-009-0101-5
|
[11] |
Ho C H, Kim J H, Kim H S, et al. 2005. Possible influence of the Antarctic Oscillation on tropical cyclone activity in the western North Pacific. Journal of Geophysical Research: Atmospheres, 110(D19): D19104. doi: 10.1029/2005JD005766
|
[12] |
Ogi M, Tachibana Y, Yamazaki K. 2003. Impact of the wintertime North Atlantic Oscillation (NAO) on the summertime atmospheric circulation. Geophysical Research Letters, 30(13): 1704. doi: 10.1029/2003GL017280
|
[13] |
Rayner N A, Parker D, Horton E B, et al. 2003. Global analyses of sea surface temperature, sea ice, and night marine air temperature since the late nineteenth century. Journal of Geophysical Research: Atmospheres, 108(D4): 4407. doi: 10.1029/2002JD002670
|
[14] |
Sun Jianqi, Wang Huijun. 2012. Changes of the connection between the summer North Atlantic Oscillation and the East Asian summer rainfall. Journal of Geophysical Research: Atmospheres, 117(D8): 8110. doi: 10.1029/2012JD017482
|
[15] |
Sun Jianqi, Wang Huijun, Yuan Wei. 2008. Decadal variations of the relationship between the summer North Atlantic Oscillation and middle East Asian air temperature. Journal of Geophysical Research: Atmospheres, 113(D15): D15107. doi: 10.1029/2007JD009626
|
[16] |
Sung M K, Kwon W T, Baek H J, et al. 2006. A possible impact of the North Atlantic Oscillation on the East Asian summer monsoon precipitation. Geophysical Research Letters, 33(21): L21713. doi: 10.1029/2006GL027253
|
[17] |
Tian Baoqiang, Fan Ke. 2012. Relationship between the late spring NAO and summer extreme precipitation frequency in the middle and lower reaches of the Yangtze River. Atmospheric and Oceanic Science Letters, 5(6): 455–460. doi: 10.1080/16742834.2012.11447038
|
[18] |
Wallace J M. 2000. North Atlantic Oscillation/annular mode: two paradigms-one phenomenon. Quarterly Journal of the Royal Meteorological Society, 126(564): 791–805. doi: 10.1256/smsqj.56401
|
[19] |
Wang Huijun, Fan Ke. 2007. Relationship between the Antarctic Oscillation in the western North Pacific typhoon frequency. Chinese Science Bulletin, 52(4): 561–565. doi: 10.1007/s11434-007-0040-4
|
[20] |
Wang Huijun, Sun Jianqi, Fan Ke. 2007. Relationships between the North Pacific Oscillation and the typhoon/hurricane frequencies. Science in China Series D: Earth Sciences, 50(9): 1409–1416. doi: 10.1007/s11430-007-0097-6
|
[21] |
Wu Bingyi, Huang Ronghui. 1999. Effects of the extremes in the North Atlantic oscillation on east asia winter monsoon. Chinese Journal of Atmospheric Sciences (in Chinese), 23(6): 641–651
|
[22] |
Wu Renguang, Kinter III J L, Kirtman B P. 2005. Discrepancy of interdecadal changes in the Asian region among the NCEP-NCAR reanalysis, objective analyses, and observations. Journal of Climate, 18(15): 3048–3067. doi: 10.1175/JCLI3465.1
|
[23] |
Wu Zhiwei, Wang Bin, Li Jianping, et al. 2009. An empirical seasonal prediction model of the East Asian summer monsoon using ENSO and NAO. Journal of Geophysical Research Atmospheres, 114(D8): D18120. doi: 10.1029/2009JD011733
|
[24] |
Wu Liang, Wen Zhiping, Huang Ronghui, et al. 2012. Possible linkage between the monsoon trough variability and the tropical cyclone activity over the western North Pacific. Monthly Weather Review, 140(1): 140–150. doi: 10.1175/MWR-D-11-00078.1
|
[25] |
Wu Renguang, Yang Song, Liu Shi, et al. 2011. Northeast China summer temperature and North Atlantic SST. Journal of Geophysical Research: Atmospheres, 116(D16): D16116. doi: 10.1029/2011JD015779
|
[26] |
Yang Song, Lau K M, Kim K M. 2002. Variations of the east Asian jet stream and Asian–Pacific–American winter climate anomalies. Journal of Climate, 15(3): 306–325. doi: 10.1175/1520-0442(2002)015<0306:VOTEAJ>2.0.CO;2
|
[27] |
Zhan Ruifen, Wang Yuqing, Lei Xiaotu. 2011. Contributions of ENSO and east Indian Ocean SSTA to the interannual variability of Northwest Pacific tropical cyclone frequency. Journal of Climate, 24(2): 509–521. doi: 10.1175/2010JCLI3808.1
|
[28] |
Zhang Hongjie, Wu Liang, Huang Ronghui. 2018. Possible impacts of two types of El Niño events on the western North Pacific monsoon trough and tropical cyclogenesis. Climatic and Environmental Research (in Chinese), 23(2): 150–160
|
[29] |
Zhou Botao, Cui Xuan. 2014. Interdecadal change of the linkage between the North Atlantic Oscillation and the tropical cyclone frequency over the western North Pacific. Science China Earth Sciences, 57(9): 2148–2155. doi: 10.1007/s11430-014-4862-z
|
[30] |
Zhou Botao, Cui Xuan, Zhao Ping. 2008. Relationship between the Asian-Pacific Oscillation and the tropical cyclone frequency in the western North Pacific. Science in China Series D: Earth Sciences, 51(3): 380–385. doi: 10.1007/s11430-008-0014-7
|
[31] |
Zhou Qun, Wei Lixin, Zhang Runyu. 2019. Influence of Indian Ocean Dipole on tropical cyclone activity over western North Pacific in boreal autumn. Journal of Ocean University of China, 18(4): 795–802. doi: 10.1007/s11802-019-3965-8
|
[32] |
Zhou Qun, Zhang Runyu. 2018. Characteristic of tropical cyclone activity over the western North Pacific in July 2017 and its link to the Arctic Oscillation. Marine Forecasts (in Chinese), 35(4): 1–7
|
[33] |
Zuo Jinqing, Li Weijing, Ren Hongli, et al. 2012. Change of the relationship between the spring NAO and East Asian summer monsoon and its possible mechanism. Chinese Journal of Geophysics, 55(1): 23–34. doi: 10.1002/cjg2.1697
|
1. | Apoorva Bamal, Md Galal Uddin, Agnieszka I. Olbert. Harnessing machine learning for assessing climate change influences on groundwater resources: A comprehensive review. Heliyon, 2024, 10(17): e37073. doi:10.1016/j.heliyon.2024.e37073 | |
2. | Liang Zhou, Rumeng Hao, Mingchen Li, et al. Spatio-temporal patterns of intense tropical cyclones in the Western North Pacific over the past 1600 years. Marine Geology, 2024, 471: 107283. doi:10.1016/j.margeo.2024.107283 | |
3. | JaeWon Choi, Il‐Ju Moon, Boo‐Keun Khim. A possible relationship between Changma and tropical cyclone genesis frequency in the western North Pacific linked to the North Atlantic Oscillation. International Journal of Climatology, 2023, 43(3): 1367. doi:10.1002/joc.7920 | |
4. | Leying Zhang, Xiting Yang, Jiuwei Zhao. Impact of the Spring North Atlantic Oscillation on the Northern Hemisphere Tropical Cyclone Genesis Frequency. Frontiers in Earth Science, 2022, 10 doi:10.3389/feart.2022.829791 |