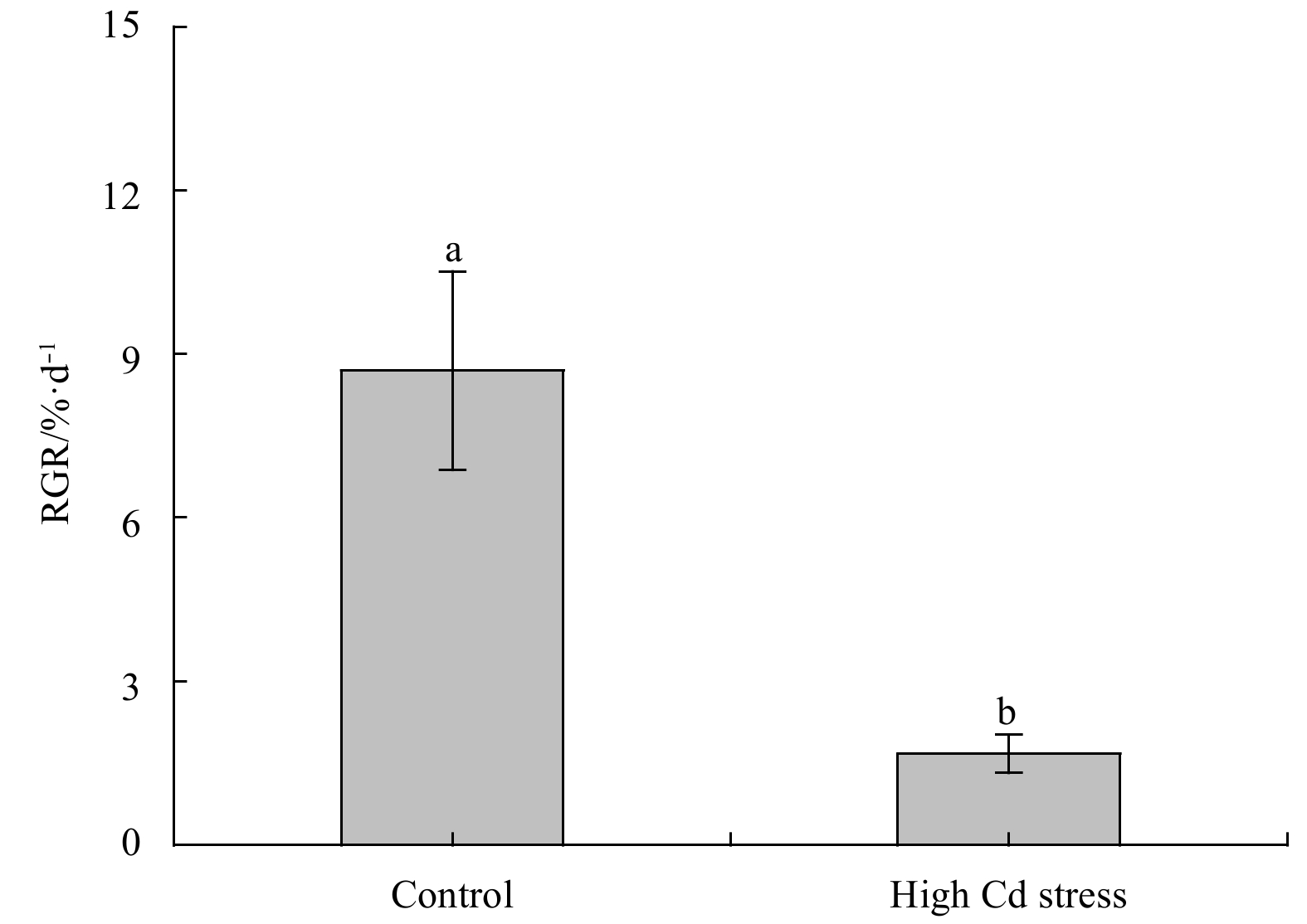
Citation: | Tiantian Zhang, Minheng Hong, Mingjiang Wu, Binbin Chen, Zengling Ma. Oxidative stress responses to cadmium in the seedlings of a commercial seaweed Sargassum fusiforme[J]. Acta Oceanologica Sinica, 2020, 39(10): 147-154. doi: 10.1007/s13131-020-1630-0 |
Heavy metal contamination caused by anthropogenic activities is one of the major worldwide environmental problems affecting water, soil, plant, animal and human health (Moreno-Jiménez et al., 2016; Du et al., 2018). Due to the potential risk to human health via the food chain (Xie et al., 2015), there has been an explosion in research on heavy metals and their effects on plant growth and development, and more specifically the tolerance of plants to Cd stress. Cd, a typical heavy metal, is commonly used in electroplating manufacturing, pigments, and the battery industry (Ruangsomboon and Wongrat, 2006). The element is non-essential for plant growth, except for some phytoplankton species, and is mostly toxic even at low concentration (Lee and Morel, 1995; Hall, 2002; Xu et al., 2007; Hernández-Candelario et al., 2019).
High Cd stress results in visible Cd toxicity symptoms in many plants, including stunted growth, chlorosis, necrosis, root browning, and even death (He et al., 2017). Cd stress could increase reactive oxygen species (ROS), and induce an oxidative stress reaction, causing toxic effects. Oxygen stress caused by Cd also affects the antioxidant system in plants. The antioxidant defense system includes the non-enzymatic antioxidants such as glutathione (GSH) and ascorbic acid (Vc), and the enzymatic antioxidants such as peroxidase (POD), superoxide dismutase (SOD), and catalase (CAT) (Li et al., 2015). Vc, GSH, vitamin E and carotenoids, and ascorbic acid-glutathione are main antioxidants in plants. There were interspecific differences in plants affected by high Cd stress. For example, when grown at high Cd levels, the activities of POD, SOD, and CAT in Atractylodes lancea were firstly increased followed by decreasing (Sun et al., 2018); while activities of SOD, POD and CAT in shoots and roots of rice (Oryza sp.) were decreased (Shao et al., 2004). In addition, Cd stress exhibits influences on the antioxidant enzyme system and the photosynthetic system in some higher plants, reducing the net photosynthetic rate (Pn) (Guo et al., 2019). Some plants could also accumulate Cd from their growth environment; in the case of edible plants, this poses a high risk to human health through the food chain (Li et al., 2015).
Cd contamination also has adverse effects on marine algae. In coastal regions, marine algae are important primary producers, providing shelter and substrate for a variety of marine animals and epiphytic algae (Ramus, 1992; Zhu et al., 2011). It is reported that cadmium treatment decreased the concentration of chlorophyll, soluble protein, and soluble phenol in Scenedesmus quadricauda (Kováčik et al., 2011). While it was demonstrated that some macroalgae, such as Gracilaria lemaneiformis, had a certain resistance to low-concentration Cd stress, and this resistance was affected by exposure time and Cd concentration; phosphate and EDTA could also increase a degree of resistance in G. lemaneiformis to Cd stress (Yu et al., 2007). Macroalgae, as well as higher plants, can resist toxic effects through protective mechanisms including antioxidants and antioxidant enzymes (Collén et al., 2003; Contreras et al., 2005). The Cd resistance and Cd biosorption capacity endowed some algae species potential biosorbents for metal sequestering and could be useful for bioremediation of Cd pollution (Patrón-Prado et al., 2011).
Sargassum fusiformis (Phaeophyceae) is a commercial brown seaweed distributed along the Northwest coast of the Pacific Ocean. The seaweed is composed of anchors, stems, leaves, bladders and reproductive tissue, and possesses a variety of biologically active antioxidant and anticoagulant components. This alga is consumed as a marine vegetable or medicinal herb in countries of Southeast Asia, such as Korea, Japan and China (Zou, 2005). Previous researches suggested that Cd could destroy the photosynthetic structure in S. fusiforme and thus influence its physiological processes, such as photosynthesis (Zhu et al., 2011) and protein expression (Zhang et al., 2015). However, there were very few studies focusing on oxidative stress in S. fusiforme under high Cd stress.
As the intensity of heavy metal pollution, increased cadmium concentration in seawater will have an impact on the culture of economic algae S. fusiforme, especially in its seedling stage. In this research, S. fusiforme seedlings were collected and cultured under two different Cd levels: natural seawater and high Cd stress. The antioxidant substances and activities of related enzyme were analyzed, aiming to investigate if inorganic cadmium stress has oxidative stress on S. fusiforme (Phaeophyceae) seedlings.
Young seedlings of Sargassum fusiforme were collected from the Luxi Island of Wenzhou, Zhejiang Province, southern China in May 2018. Healthy algal thalli were selected after washing off the visible epiphytes and accumulated sediments with seawater, and then were pre-cultured for 2 d under 100 μmol photons m–2 s–1 illumination by fluorescent tubes for 12:12 (light:dark) photoperiod at 20.3(±1)°C. Culture medium was composed of sterile seawater supplied with additional 200 μmol/L
For this experiment, S. fusiforme seedlings were cultured under two inorganic Cd levels, including the natural seawater (control) and Cd enrichment (treatment). Concentration of Cd2+ in natural seawater was ~0.9 μmol/L. As compared to the control, treated thalli were cultured in the sterile seawater that enriched with 100 μmol/L CdCl2. Approximately 30 g thalli were cultured in 10-L tank as the density. Culture medium was renewed every second day. Each level had three replicates. Other culture conditions were maintained as the pre-culture. After 10 d of indoor culture, thalli were collected and reserved under –20°C condition until physiological and biochemical tests were performed.
Relative growth rates (RGR, %/d) were calculated from three replicates using the following equation: RGR = ln (X1/X0)/(T1 – T0)×100, where X0 referred to the fresh weight (Fw) of the cultured thalli at the T0 days and X1 referred to the Fw at the T1 days.
Approximately 0.5 g (Fw) of each sample was used to determine the net photosynthetic oxygen evolution rate (Pn) at 400 μmol photons m–2 s–1 and dark O2 consumed rate (dark respiration, Rd) by a Clark-type oxygen electrode (YSI Model 5300; Yellow Springs Instrument Co., OH, USA) with a water jacket connected to a cooling circulator (Cole Parmer, USA). The temperature was maintained at 20°C. Light intensity was provided by a halogen lamp. The samples of algae were allowed to acclimate to the electrode cuvette environment for 10 min before measurement. Values of Pn and Rd were all expressed in (μmol O2)/(g·h) of Fw.
Approximately 100 mg of fresh tissues was ground in 10 mL of absolute methanol and extracted for 24 h at 4°C in the dark. This extract was centrifuged at 8000 r/min for 10 min, and then the supernatant was used to determine the amounts of chlorophyll a (Chl a) and carotenoid (Caro) using an ultraviolet spectrophotometer (UV-1800; Shimadzu, Kyoto, Japan). Content of Chl a and Caro was estimated according to Porra (2002) and Parsons and Strickland (1963), respectively.
Soluble protein (SP) content was determined by xylene brilliant cyanin G. About 0. 2 g (Fw) sample was homogenated in the mortar with 10 mL saline. The homogenate was centrifuged at 2500 r/min for 15 min. A total of 1 mL supernatant was transferred into the test tube, then added with 3 mL xylene brilliant cyanin G solution. After 5 min standing, absorbance at 595 nm was recorded by ultraviolet spectrophotometer (UV-1800; Shimadzu, Kyoto, Japan) and calculated according to the absorbance value standard curve (Bradford, 1976).
Inorganic Cd uptake rates (V) of the seedlings in 48 h during one seawater renewal period, were probed using inductively coupled plasma atomic emission spectrometry (PerkinElmer Optima 7300V ICP USA). Each seawater medium was filtered by 0.45 μm cellulose filter membrane before analyzed.
Seedling samples were dipped in 30% H2O2 to eluting the epiphytes if any, and washed by deionized water, then they were dried at 40°C. Cd accumulation in young seedlings was performed using inductively coupled plasma mass spectrometry (NexION 1000 ICP-MS, PerkinElmer, USA). Specifically, Cd accumulations in stem and leaf (air sac) were determined separately.
Approximately 200 mg of fresh tissue was homogenized in 10 mL of phosphate buffer. The homogenate was centrifuged at 5000 r/min at 4°C for 10 min. The supernatant was stored at 4°C for analysis of the antioxidation activities in S. fusiforme seedlings. The total superoxide dismutase (T-SOD) was measured by the photochemical method (Giannopolitis and Ries, 1977) with minor modification. One-unit SOD activity was defined as the amount of enzyme required for a 50% inhibition of the rate of NBT (r-nitro blue tetrazolium chloride) reduction that was measured at 550 nm. CAT activity was measured by the spectrophotometric method based on the decomposition of H2O2 in 50 mmol/L phosphate buffer and monitored at 234 nm (Aebi, 1983).
MDA content that accumulates in algal following extreme stress (Khatun et al., 2008; Zhu et al., 2011), was measured using a thiobarbituric acid (TBA) reaction to indicate the lipid peroxidation (Heath and Packer, 1968). The absorbance of the supernatant at 532 nm was recorded and corrected for unspecific turbidity by subtracting the value at 600 nm. Concentration of MDA was calculated using the extinction coefficient of 155 mmol/(L·cm). Activities of POD, GR, CAT, and contents of Vc, DHA, GSH, were analyzed by colorimetric assay kits (Jiancheng Bioengineering Institute, Nanjing, China) following the manufacturer’s instructions.
Statistical analysis was carried out using a statistical package Origin 9.0 (OriginLab, USA). One-way analysis of variance (ANOVA) and the Tukey’s test were used to analyze differences between treatment and control. A P-value of 0.05 was considered statistically significant.
The morphology and RGR were displayed in Fig. 1. As compared to the control, Cd stress significantly depressed growth of S. fusiforme seedlings, lowering RGR over 80% (F1, 4=42.21, P<0.01). Under high Cd level condition, seedlings generally appeared smaller size and darker color as compared to the control (Fig. 2a).
As displayed in Fig. 3, value of Pn in S. fusiforme seedlings was significantly lowered by high Cd treatment (F1, 4=14.67, P<0.05), while the increase of Rd was not significant under the high Cd level (F1, 4=4.69, P=0.10). However, values of Rd/Pn were significantly increased by high Cd stress (F1, 4=15.26, P<0.05).
Contents of both the Chl a and Caro were significantly decreased by the high Cd treatment (Chl a: F1, 4=26.07, P<0.01; Caro: F1, 4=15.26, P<0.05) (Fig. 4a). However, SP in S. fusiforme seedlings was significantly elevated by high Cd treatment (Fig. 4b, F1, 4=28.86, P<0.01).
When growing at high Cd (Fig. 5), the uptake rate (V) in seedlings was higher than the control outstandingly (F1, 4=146.35, P<0.01). Correspondingly, Cd contents accumulated in the thalli growing at high Cd treatment, including both the S. fusiforme stem and leaf (air sac), were much higher than the control (stem: F1, 4=1475.48, P<0.01; leaf: F1,4=1100.74, P<0.01). Specifically, under high Cd treatment, Cd accumulated in stem was higher than in leaf (F1,4=104.85, P<0.01); while there was no significant difference between the stem and leaf growing at the control condition (F1, 4=1.81, P=0.25).
After 10 d of indoor culture, DHA content and GR activity were significantly lower in the metal-treated algae than the controls (DHA: F1, 4=204.41, P<0.01; GR: F1, 4=17.58, P<0.05). However, high Cd culture significantly increased the activities of POD and T-SOD by about 75.9% and 11.5%, and also the contents of Vc and GSH by about 26% and 110%, respectively (P<0.05) (Fig. 6).
Figure 7 showed a non-significantly influence of high Cd stress on MDA contents in S. fusiforme seedlings (F1, 4=6.11, P=0.07). While the CAT activity was significantly increased under high Cd culture (F1, 4=26.31, P<0.01).
Cadmium (Cd), a common heavy metal pollutant in the aquatic environment, is generally toxic to plants, leading to growth inhibition and biomass reduction (Rizwan et al., 2016; Lü et al., 2018). In the present study, high Cd stress similarly exhibited significant inhibition on the growth of S. fusiforme seedlings and increased algal thalli color burn, especially the older leaves. Inhibited growth was also reported in mature S. fusiforme thalli (Zhang et al., 2015), and other algae species such as Picocystis sp. (Rihab et al., 2017). Under high Cd stress, Cd accumulated in S. fusiforme seedlings and fundamentally impeded their physiological processes, including photosynthesis and antioxidation mechanisms, resulting in algal growth inhibition, which was consistent with the previous study in Sargassum cymosum (Costa et al., 2017).
Although Cd-ions are not essential for algal growth, they can be absorbed via facilitated diffusion and active transport (Wójcik et al., 2005). Thus, the Cd uptake rate under high Cd conditions is much higher than in the control group. Seedlings grown under a high Cd level exhibited higher Cd accumulations in both the stems and the leaves; this might have contributed to the darker color of the seedlings. The S. fusiforme stem accumulated more Cd than the leaves, which was a result of the higher growth and metabolic transport rates in the seedling leaves compared to the stem tissue.
Cd accumulation in S. fusiforme seedlings significantly affected the activities of antioxidants and antioxidizes. Under Cd stress, algae produce ROS to destruct the biological macromolecules, leading to reduced or inactive enzymes, and inducing the synthesis or activation of ROS-related clearance enzymes (Zhao et al., 2008; Souza et al., 2012), although it may also induce redox signals to regulate physiological processes (Kacperska, 2004). The large number of free radicals produced by high Cd stress promotes lipid peroxidation and causes severe damage to the biomembranes in plant cells, including cell membranes, and chloroplasts (Panda and Choudhury, 2005).
Under the high Cd stress generated in this study, the activities of SOD, CAT and POD in S. fusiforme seedlings increased significantly. In order to decrease oxidative damage, several metabolites and enzymes including SOD, ascorbate peroxidase (APX) and CAT were functionally activated (Xie et al., 2015). When exposed to high Cd stress, S. fusiforme seedlings activated the first defense line of SOD to resist the oxidative damage. However, the seedlings increased CAT activity. Although Cd ions destroyed photosynthetic pigments in seedlings, H2O2 content was reduced by photosynthetic electron transport reaction. The seedlings activated POD, a terminal containing iron oxidase, which oxidizes phenols in the presence of H2O2 and disseminating H2O2 from plant cells, thereby preventing the free radicals from attacking membrane lipids. Under adverse conditions, increasing POD activity is a common mechanism to remove excess ROS in plants, while the POD activity typically decreased when stress exceeded the plants’ ability to regulate (Yu et al., 2007).
Among the array of antioxidant pathways, the AsA-GSH cycle is the most important circulatory system. The AsA-GSH cycle is mainly present in the cytoplasm and chloroplasts of algal cells to disseminate H2O2. The primary regulators of this cycle consist of non-enzymatic antioxidants (Tian and Yu, 2009). The experiment in this study showed that the concentrations of GSH and Vc increased significantly under high Cd levels, whereas the DHA concentration and the GR activity decreased. The synthesis of GSH was enhanced due to the regulation of S-adenosylmethionine synthetase (SAMS) and GS, both involved in GSH synthesis (Xiao et al., 2015). GSH is a major intracellular antioxidant and a precursor of phytochelatins, which can form a chelate with Cd2+ and reduce metal ion toxicity (Wójcik and Tukiendorf, 2011). A decrease in the GR activity also lowered the reduction reaction of GSH. Additionally, as a regulator of ascorbate-glutathione, GSH can accelerate the whole cycle of antioxidant system. This effect was also demonstrated by a significant increase of Vc and a decrease of DHA concentrations. However, under high Cd stress, there was no significant increase in MDA compared to the control group. The observation was consistent with the results in higher plants as reported by Li et al. (2015). These reactions mainly result from the effective antioxidant system, including enhanced CAT activity, to remove active oxygen.
Inhibited growth under high Cd stress was also a result of restricted photosynthesis. Under high Cd stress, Pn and photosynthetic pigments in S. fusiforme seedlings were reduced. This was consistent with the previous studies on Gracilaria tenuistipitata (Simioni et al., 2015), and Gracilaria domingensis (Dos Santos et al., 2012). Earlier studies showed that under high Cd stress, S. fusiforme reduced photosynthetic efficiency by down-regulating the synthesis of key enzymes such as 1, 5-bisphosphate ribulose carboxylase/oxygenase (Rubisco) and phosphoglycerate kinase (PGK) (Zhang et al., 2015). Thus, the decreased photosynthesis rate in S. fusiforme seedlings under high Cd level resulted from the decreased activity and synthesis of enzymes and the reduced photosynthetic pigmentation. As a result of the reduced photosynthetic pigments and inhibited growth, the proportion of other pigments such as xanthophyll increased. Similarly, the amount of blocked Cd in the cytoderm of old and slow-growing tissues in the thalli expanded (Wójcik et al., 2005), and the color of S. fusiforme thalli deepened.
The lower pigment content in this study also indicated that photosynthetic pigments were one of the targets of Cd-ions. Photosynthetic pigments and devices of plants were considered the main victims of heavy metal pollution (Xia et al., 2004; Baumann et al., 2009). The reduction of pigments might also result from the destruction of the chloroplasts, the pigment synthesis site. Chloroplasts play a major role in the regulation of ‘redox homeostasis’ in plant cells and are the first to be affected by oxidative stress (Kacperska, 2004). As demonstrated in Chlorella pyrenoidosa (Wang et al., 2013), Cd stress impairs the chloroplasts structure and function and interferes with their stable bond to proteins, thereby damages the photosynthetic apparatus (Küpper et al., 2007). Moreover, Cd-ions can bind to the mercaptan group in the enzyme activity center and replace the essential element in the metalloproteins, such as Mg2+ in the chlorophyll molecule. This replacement lowers the light-harvesting ability of chlorophyll (Küpper et al., 1998) and affects the activity of enzymes related to chlorophyll synthesis (Stobart et al., 1985). Consequently, the synthesis of chlorophyll, which contributes to the light energy capture for photosynthesis in S. fusiforme seedlings, was inhibited as well as the photosynthesis.
Under high Cd stress, the concentration of soluble protein was significantly higher than in the control group, indicating that the protein expression process in the seedlings was also affected. Due to the high Cd stress, a strong increase in proteins involved in glycolysis by the TCA cycle (Kieffer et al., 2009) and the antioxidant reaction (Yu et al., 2007) were activated, which synthesized amount of protein, including kinds of enzymes. When the algae are subjected to Cd stress, many mechanisms were involved in suppressing the damages, such as generating Cd resistance proteins and activating Cd transport. The resistance mechanisms in algae are energy-intensive. This study also found that Rd/Pn of S. fusiforme seedlings increased significantly under high Cd stress, indicating the increased energy consumption to maintain normal metabolisms.
In conclusion, high Cd stress caused Cd accumulation in the stem and leaves of S. fusiforme seedlings. Sargassum fusiforme seedlings could active a series of resistance mechanism to defend against high Cd stress (as shown in Fig. 2b), such as increasing the antioxidant activities of POD, SOD, Vc, and the AsA-GSH cycle to disseminate H2O2 and to maintain a healthy metabolism in the algal cells. High levels of Cd in the environment are confirmed to have toxic effects on commercial S. fusiforme seedlings. The excessive Cd significantly inhibited the photosynthesis and reduced the photosynthetic pigments in the seedlings, resulting in inhibited growth and deep morphological color, especially in the stems. Thus, Cd pollution negatively influences the S. fusiforme culture industry, and the accumulated Cd can enter human bodies via the edible seaweed.
We appreciate all staffs for their helpful assistance during this research.
[1] |
Aebi H E. 1983. Catalase. In: Bergmeyer U S, ed. Methods of Enzymatic Analysis. 3rd ed. Weinheim: Verlag-Chemie
|
[2] |
Baumann H A, Morrison L, Stengel D B. 2009. Metal accumulation and toxicity measured by PAM—Chlorophyll fluorescence in seven species of marine macroalgae. Ecotoxicology and Environmental Safety, 72(4): 1063–1075. doi: 10.1016/j.ecoenv.2008.10.010
|
[3] |
Bradford M M. 1976. A rapid and sensitive method for the quantitation of microgram quantities of protein utilizing the principle of protein-dye binding. Analytical Biochemistry, 72(1–2): 248–254. doi: 10.1016/0003-2697(76)90527-3
|
[4] |
Collén J, Pinto E, Pedersén M, et al. 2003. Induction of oxidative stress in the red macroalga Gracilaria tenuistipitata by pollutant metals. Archives of Environmental Contamination and Toxicology, 45(3): 337–342
|
[5] |
Contreras L, Moenne A, Correa J A. 2005. Antioxidant responses in Scytosiphon lomentaria (Phaeophyceae) inhabiting copper-enriched coastal environments. Journal of Phycology, 41(6): 1184–1195. doi: 10.1111/j.1529-8817.2005.00151.x
|
[6] |
Costa G B, Simioni C, Pereira D T, et al. 2017. The brown seaweed Sargassum cymosum: changes in metabolism and cellular organization after long-term exposure to cadmium. Protoplasma, 254(2): 817–837. doi: 10.1007/s00709-016-0992-9
|
[7] |
Dos Santos R W, Schmidt É C, Martins R D P, et al. 2012. Effects of cadmium on growth, photosynthetic pigments, photosynthetic performance, biochemical parameters and structure of chloroplasts in the Agarophyte Gracilaria domingensis (Rhodophyta, Gracilariales). American Journal of Plant Sciences, 3(8): 1077–1084. doi: 10.4236/ajps.2012.38129
|
[8] |
Du Hong, Liang Honghao, Jiang Yang, et al. 2018. Proteome responses of Gracilaria lemaneiformis exposed to lead stress. Marine Pollution Bulletin, 135: 311–317. doi: 10.1016/j.marpolbul.2018.07.030
|
[9] |
Giannopolitis C N, Ries S K. 1977. Superoxide dismutases: I. Occurrence in higher plants. Plant Physiology, 59(2): 309–314. doi: 10.1104/pp.59.2.309
|
[10] |
Guo Jiajia, Qin Shiyu, Rengel Z, et al. 2019. Cadmium stress increases antioxidant enzyme activities and decreases endogenous hormone concentrations more in Cd-tolerant than Cd-sensitive wheat varieties. Ecotoxicology and Environmental Safety, 172: 380–387. doi: 10.1016/j.ecoenv.2019.01.069
|
[11] |
Hall J L. 2002. Cellular mechanisms for heavy metal detoxification and tolerance. Journal of Experimental Botany, 53(366): 1–11
|
[12] |
He Shanying, Yang Xiaoe, He Zhenli, et al. 2017. Morphological and physiological responses of plants to cadmium toxicity: a review. Pedosphere, 27(3): 421–438. doi: 10.1016/S1002-0160(17)60339-4
|
[13] |
Heath R L, Packer L. 1968. Photoperoxidation in isolated chloroplasts: I. Kinetics and stoichiometry of fatty acid peroxidation. Archives of Biochemistry and Biophysics, 125(1): 189–198. doi: 10.1016/0003-9861(68)90654-1
|
[14] |
Hernández-Candelario I D C, Lares M L, Camacho-Ibar V F, et al. 2019. Dissolved cadmium and its relation to phosphate in the deep region of the Gulf of Mexico. Journal of Marine Systems, 193: 27–45. doi: 10.1016/j.jmarsys.2019.01.005
|
[15] |
Kacperska A. 2004. Sensor types in signal transduction pathways in plant cells responding to abiotic stressors: do they depend on stress intensity?. Physiologia Plantarum, 122(2): 159–168. doi: 10.1111/j.0031-9317.2004.00388.x
|
[16] |
Khatun S, Ali M B, Hahn E J, et al. 2008. Copper toxicity in Withania somnifera: Growth and antioxidant enzymes responses of in vitro grown plants. Environmental and Experimental Botany, 64(3): 279–285. doi: 10.1016/j.envexpbot.2008.02.004
|
[17] |
Kieffer P, Schröder P, Dommes J, et al. 2009. Proteomic and enzymatic response of poplar to cadmium stress. Journal of Proteomics, 72(3): 379–396. doi: 10.1016/j.jprot.2009.01.014
|
[18] |
Kováčik J, Klejdus B, Štork F, et al. 2011. Comparison of methyl jasmonate and cadmium effect on selected physiological parameters in scenedesmus quadricauda (chlorophyta, chlorophyceae). Journal of Phycology, 47(5): 1044–1049. doi: 10.1111/j.1529-8817.2011.01027.x
|
[19] |
Küpper H, Küpper F, Spiller M. 1998. In situ detection of heavy metal substituted chlorophylls in water plants. Photosynthesis Research, 58(2): 123–133. doi: 10.1023/A:1006132608181
|
[20] |
Küpper H, Parameswaran A, Leitenmaier B, et al. 2007. Cadmium-induced inhibition of photosynthesis and long-term acclimation to cadmium stress in the hyperaccu-mulator Thlaspi caerulescens. New Phytologist, 175(4): 655–674. doi: 10.1111/j.1469-8137.2007.02139.x
|
[21] |
Lee J G, Morel F M M. 1995. Replacement of zinc by cadmium in marine phytoplankton. Marine Ecology Progress Series, 127: 305–309. doi: 10.3354/meps127305
|
[22] |
Li Ziwei, Yang Ye, Cui Xiuming, et al. 2015. Physiological response and bioaccumulation of Panax notoginseng to cadmium under hydroponic. China Journal of Chinese Materia Medica (in Chinese), 40(15): 2903–2908
|
[23] |
Lü Fang, Ding Gang, Liu Wei, et al. 2018. Comparative study of responses in the brown algae Sargassum thunbergii to zinc and cadmium stress. Journal of Oceanology and Limnology, 36(3): 933–941. doi: 10.1007/s00343-018-6334-3
|
[24] |
Moreno-Jiménez E, Fernández J M, Puschenreiter M, et al. 2016. Availability and transfer to grain of As, Cd, Cu, Ni, Pb and Zn in a barley agri-system: Impact of biochar, organic and mineral fertilizers. Agriculture, Ecosystems & Environment, 219: 171–178
|
[25] |
Panda S K, Choudhury S. 2005. Chromium stress in plants. Brazilian Journal of Plant Physiology, 17(1): 95–102. doi: 10.1590/S1677-04202005000100008
|
[26] |
Parsons T R, Strickland J D H. 1963. Discussion of spectrophotometric determination of marine plant pigments, with revised equations for ascertaining chlorophylls and carotenoids. Journal of Marine Research, 21(3): 155–163
|
[27] |
Patrón-Prado M, Casas-Valdez M, Serviere-Zaragoza E, et al. 2011. Biosorption capacity for cadmium of brown seaweed Sargassum sinicola and Sargassum lapazeanum in the gulf of California. Water, Air, & Soil Pollution, 221(1–4): 137–144
|
[28] |
Porra R J. 2002. The chequered history of the development and use of simultaneous equations for the accurate determination of chlorophylls a and b. Photosynthesis Research, 73(1–3): 149–156
|
[29] |
Ramus J. 1992. Productivity of seaweeds. In: Falkowski P G, Woodhead A D, eds. Primary Productivity and Biogeochemical Cycles in the Sea. New York: Plenum Press
|
[30] |
Rihab B A, Sabrine B O, Lina C, et al. 2017. Cadmium effect on physiological responses of the tolerant chlorophyta specie picocystis sp. isolated from tunisian wastewaters. Environmental Science and Pollution Research, 24(2): 1803–1810. doi: 10.1007/s11356-016-7950-0
|
[31] |
Rizwan M, Ali S, Abbas T, et al. 2016. Cadmium minimization in wheat: a critical review. Ecotoxicology and Environmental Safety, 130: 43–53. doi: 10.1016/j.ecoenv.2016.04.001
|
[32] |
Ruangsomboon S, Wongrat L. 2006. Bioaccumulation of cadmium in an experimental aquatic food chain involving phytoplankton (Chlorella vulgaris), zooplankton (Moina macrocopa), and the predatory catfish Clarias macrocephalus×C. gariepinus. Aquatic Toxicology, 78(1): 15–20. doi: 10.1016/j.aquatox.2006.01.015
|
[33] |
Shao Guosheng, Muhammad J H, Zhang Xiufu, et al. 2004. Effects of cadmium stress on plant growth and antioxidative enzyme system in different rice genotypes. Chinese Journal of Rice Science (in Chinese), 8(3): 239–244
|
[34] |
Simioni C, Schmidt É C, Rover T, et al. 2015. Effects of cadmium metal on young gametophytes of Gelidium floridanum: metabolic and morphological changes. Protoplasma, 252(5): 1347–1359. doi: 10.1007/s00709-015-0768-7
|
[35] |
Souza O P, Ferreira R L, Pires N R X, et al. 2012. Algae of economic importance that accumulate cadmium and lead: a review. Revista Brasileira de Farmacognosia, 22(4): 825–837. doi: 10.1590/S0102-695X2012005000076
|
[36] |
Stobart A K, Griffiths W T, Ameen-Bukhari I, et al. 1985. The effect of Cd2+ on the biosynthesis of chlorophyll in leaves of barley. Physiologia Plantarum, 63(3): 293–298. doi: 10.1111/j.1399-3054.1985.tb04268.x
|
[37] |
Sun Yongdi, Chao Jianguo, Gu Wei, et al. 2018. Effect of cadmium stress on physiological and biochemical characteristics of Atractylodes lancea. Plant Physiology Journal (in Chinese), 54(12): 1857–1864
|
[38] |
Tian Jiyuan, Yu Juan. 2009. Changes in ultrastructure and responses of antioxidant systems of algae (Dunaliella salina) during acclimation to enhanced ultraviolet-B radiation. Journal of Photochemistry and Photobiology B: Biology, 97(3): 152–160. doi: 10.1016/j.jphotobiol.2009.09.003
|
[39] |
Wang Shuzhi, Zhang Daoyong, Pan Xiangliang. 2013. Effects of cadmium on the activities of photosystems of Chlorella pyrenoidosa and the protective role of cyclic electron flow. Chemosphere, 93(2): 230–237. doi: 10.1016/j.chemosphere.2013.04.070
|
[40] |
Wójcik M, Tukiendorf A. 2011. Glutathione in adaptation of Arabidopsis thaliana to cadmium stress. Biologia Plantarum, 55(1): 125–132. doi: 10.1007/s10535-011-0017-7
|
[41] |
Wójcik M, Vangronsveld J, D’Haen J, et al. 2005. Cadmium tolerance in Thlaspi caerulescens: Ⅱ. Localization of cadmium in Thlaspi caerulescens. Environmental and Experimental Botany, 53(2): 163–171
|
[42] |
Xia J R, Li Y J, Lu J, et al. 2004. Effects of copper and cadmium on growth, photosynthesis, and pigment content in Gracilaria lemaneiformis. Bulletin of Environmental Contamination and Toxicology, 73(6): 979–986. doi: 10.1007/s00128-004-0522-x
|
[43] |
Xiao Qingtie, Wang Jingyuan, Zheng Xinyu, et al. 2015. Analysis of the differently expressed proteins in rice roots in response to cadmium stress. Acta Ecologica Sinica (in Chinese), 35(24): 8276–8283
|
[44] |
Xie Yang, Ye Shan, Wang Yan, et al. 2015. Transcriptome-based gene profiling provides novel insights into the characteristics of radish root response to Cr stress with next-generation sequencing. Frontiers in Plant Science, 6: 202
|
[45] |
Xu Yan, Tang Degui, Shaked Y, et al. 2007. Zinc, cadmium, and cobalt interreplacement and relative use efficiencies in the coccolithophore Emiliania huxleyi. Limnology and Oceanography, 52(5): 2294–2305. doi: 10.4319/lo.2007.52.5.2294
|
[46] |
Yu Jiang, Yang Yufeng, Nie Xiangping. 2007. Response of seaweed Gracilaria Lemaneiformis to Cadmium stress. Journal of Sichuan University (Engineering Science Edition) (in Chinese), 39(3): 83–90
|
[47] |
Zhang Aiqin, Xu Tao, Zou Huixi, et al. 2015. Comparative proteomic analysis provides insight into cadmium stress responses in brown algae Sargassum fusiforme. Aquatic Toxicology, 163: 1–15. doi: 10.1016/j.aquatox.2015.03.018
|
[48] |
Zhao Tianhong, Sun Jiawei, Fu Yu. 2008. Advances of research on metabolism of plant reactive oxygen species and exogenous regulation under abiotic stresses. Crops (in Chinese), (3): 10–13
|
[49] |
Zhu Xifeng, Zou Dinghui, Du Hong. 2011. Physiological responses of Hizikia fusiformis to copper and cadmium exposure. Botanica Marina, 54(5): 431–439
|
[50] |
Zou Dinghui. 2005. Effects of elevated atmospheric CO2 on growth, photosynthesis and nitrogen metabolism in the economic brown seaweed, Hizikia fusiforme (Sargassaceae, Phaeophyta). Aquaculture, 250(3–4): 726–735
|
1. | Yiqi Kuang, Donya Bao, Xingda Sheng, et al. Gradient experiment reveals physiological stress from heavy metal zinc on the economically valuable seaweed Sargassum fusiforme. Marine Environmental Research, 2025, 204: 106958. doi:10.1016/j.marenvres.2025.106958 | |
2. | Xiaojie Zuo, Xingda Sheng, Mingjiang Wu, et al. Evaluation of tissue-regenerated Sargassum fusiforme (Harvey) Setchell: Perspective of physiological and nutritional quality. Aquaculture, 2025, 600: 742216. doi:10.1016/j.aquaculture.2025.742216 | |
3. | Lin Luo, Dongya Bao, Congquan Gao, et al. Gibberellin induces rapid regeneration of juveniles from the Sargassum fusiforme holdfasts. Aquaculture, 2025. doi:10.1016/j.aquaculture.2025.742248 | |
4. | Li Liang, Lin Luo, Hangjun Wang, et al. Effects of temperature and irradiance on the growth of regenerated juveniles from Sargassum fusiforme holdfasts. Marine Biology Research, 2024, 20(1-2): 15. doi:10.1080/17451000.2024.2312900 | |
5. | Yuh Tzean, Kuang-Teng Wang, Po-Yi Lee, et al. Assessing the impact of arsenite and arsenate on Sarcodia suae: a tale of two toxicities. Ecotoxicology, 2024, 33(8): 937. doi:10.1007/s10646-024-02793-5 | |
6. | Huayan Huang, Ke Liang, Yuxian Shangguan, et al. Effect of coexisting nutrient divalent cations on cadmium transport in soil-herbal crop systems. Chemosphere, 2024, 369: 143848. doi:10.1016/j.chemosphere.2024.143848 | |
7. | Weimin Chen, Manying Sun. Acute copper stress showed toxic effects on the physiological metabolism of Ulva lactuca, a common green macroalgae. Scientific Reports, 2024, 14(1) doi:10.1038/s41598-024-76517-4 | |
8. | Rachel J. Clausing, Annalisa Falace, Gina De La Fuente, et al. Ex-situ restoration of the Mediterranean forest-forming macroalga Ericaria amentacea: Optimizing growth in culture may not be the key to growth in the field. Marine Environmental Research, 2024, 202: 106718. doi:10.1016/j.marenvres.2024.106718 | |
9. | Jianpan Xin, Sisi Ma, Hanwen Xiao, et al. Copper Accumulation and its Effect on Glyoxalase System, AsA-GSH Cycle, Antioxidase Activity in Pontederia cordota Leaves. Water, Air, & Soil Pollution, 2024, 235(6) doi:10.1007/s11270-024-07030-7 | |
10. | Lin Luo, Xiaojie Zuo, Lina Guo, et al. Effects of exogenous hormones on the regeneration of juveniles from Sargassum fusiforme holdfasts. Frontiers in Marine Science, 2023, 9 doi:10.3389/fmars.2022.1072391 | |
11. | Shuaipeng Tian, Binbin Chen, Mingjiang Wu, et al. Are there environmental benefits derived from coastal aquaculture of Sargassum fusiforme?. Aquaculture, 2023, 563: 738909. doi:10.1016/j.aquaculture.2022.738909 | |
12. | Daiane Franchesca Senhor, Marcos Vinicius Miranda Aguilar, Caroline Castro Kuinchtner, et al. Silicon-induced changes in morphophysiological and biochemical characteristics in Enterolobium contortisiliquum under cadmium stress. Rodriguésia, 2023, 74 doi:10.1590/2175-7860202374059 | |
13. | Guanfeng Pang, Lin Luo, Lina Guo, et al. Temporal and spatial changes of major endogenous phytohormones during the regeneration of juveniles from Sargassum fusiforme holdfasts. Journal of Applied Phycology, 2023, 35(6): 2995. doi:10.1007/s10811-023-03113-w | |
14. | Hyun-Hwa Lee, Jin-Sol Kim, Jun-Han Jeong, et al. Comparative Analysis of Biological Activities and Phenolic Content between Fresh and Steamed Sargassum fusiforme in Different Extraction Solvents. Applied Sciences, 2022, 12(23): 12161. doi:10.3390/app122312161 | |
15. | Shuaipeng Tian, Tingting Zheng, Mingjiang Wu, et al. Differences of photosynthesis and nutrient utilization in Sargassum fusiforme and its main epiphyte, Ulva lactuca. Aquaculture Research, 2022, 53(8): 3176. doi:10.1111/are.15830 | |
16. | Lili Xu, Lin Luo, Xiaojie Zuo, et al. Effects of temperature and irradiance on the regeneration of juveniles from the holdfasts of Sargassum fusiforme, a commercial seaweed. Aquaculture, 2022, 557: 738317. doi:10.1016/j.aquaculture.2022.738317 | |
17. | Lili Xu, Lidong Lin, Lin Luo, et al. Organic acid treatment for removal of epiphytic Ulva L. attached to Sargassum fusiforme seedlings. Aquaculture, 2022, 547: 737533. doi:10.1016/j.aquaculture.2021.737533 | |
18. | Ting Wei, Xian Li, Noman Yashir, et al. Effect of exogenous silicon and methyl jasmonate on the alleviation of cadmium-induced phytotoxicity in tomato plants. Environmental Science and Pollution Research, 2021, 28(37): 51854. doi:10.1007/s11356-021-14252-3 | |
19. | C Castro Kuinchtner, G Silva Wertonge de Oliveira, MV Miranda de Aguilar, et al. Can species Cedrela fissilis Vell. be used in sites contaminated with toxic aluminum and cadmium metals?. iForest - Biogeosciences and Forestry, 2021, 14(6): 508. doi:10.3832/ifor3890-014 |